LEGO ALMA
To illustrate how an astronomical radio interferometer works, we partly recreated the ALMA observatory out of LEGO. Our small telescopes are blind, but two microprocessors detect where they are located and also where a selectable astronomical object is in the sky. From this information, a computer calculates the image that ALMA would obtain in this arrangement.
If one changes the number of the maximum 19 LEGO telescopes or their relative position, the calculated image changes. A small image of the celestial object can be moved to different positions on the back wall. The original image of the object is displayed on the monitor, as well as the image observed through our LEGO ALMA. The more telescopes are observing and the farther and more evenly spaced the telescopes are relative to the object direction, the better the observed image will be. Each time the configuration is changed, a new image is calculated in real time. In this way, it is possible to playfully explore what we can see with a radio interferometer.
A few technical details
The sharpness of an image - whether taken through the eye, a camera lens, or a telescope - depends only on the wavelength of the detected light and the diameter of the lens: the larger the telescope, the sharper the image. The human eye, for example, has a resolving power of one minute of arc, which is 1/60 of an angular degree, or about 1/30 of the diameter of the moon. The resolution becomes proportionally better the smaller the wavelength and the larger the lens: At a wavelength of 2 cm, the 100-meter Effelsberg radio telescope (Eifel) achieves a resolution similar to that of the human eye.
Many astronomical objects are much smaller and observations require an angular resolution of one arc second (1/60 arc minute) or less, which would require larger telescopes or observing at shorter wavelengths. The fabrication accuracy of a lens or concave mirror must be better than 1/20th of the wavelength of the observed radiation, which quickly pushes the technical challenges and costs of larger telescopes to the limits of feasibility.
The interferometry trick: By cleverly combining several small telescopes, a resolving power equivalent to that of a much larger telescope can be achieved; here, it is not the diameter of the individual telescopes that is decisive, but the maximum distance between the telescopes. any distributed, small telescopes then act like a very spotty, large telescope. The poorly filled collecting surface of such an interferometer has its price: The image has a better resolution (image sharpness), but it looks filtered like through dirty and spotty glasses. Therefore, one always chooses the local arrangement of the telescopes in such a way that the information hoped for from the celestial object is optimally visible: the widest possible distribution of the telescopes to see sharp details, with the more extended structures disappearing, or vice versa. The true overall image of the source is difficult to image with an interferometer, but it offers us the perception of minute details that would never be visible with a (feasible) single telescope.
The computational cost of an interferometer is enormous. Each telescope has a receiver that constantly registers the radiation intensity and wave phase of the targeted radiation source and feeds it to a central "correlator". Separated into narrow frequency intervals, this correlator correlates (i.e. multiplies and averages) the signals of all telescopes in pairs (for the 50 ALMA telescopes this is 50*49/2=1225 pairs) and averages the measurements to a few seconds. ALMA stores about 500 megabytes of data per second. From these so-called visibilities an image of the celestial object can be calculated - as mentioned, seen through a sort of filter.
Our LEGO model also computes the observed, filtered image of a selectable object: a spiral galaxy, an S-shaped structure with embedded point sources, a geometric pattern, or onlookers captured directly by a camera.
Responsible for design and setup is Prof. Bertoldi's research group at the Argelander Institute for Astronomy at the University of Bonn, in particular Toma Badescu, Philipp Müller, Dr. Benjamin Magnelli and Dr. Stefanie Mühle.
In 2022 we supported the creation of a copy of our LEGO-ALMA by the group of Prof. Hacar at the University of Vienna.
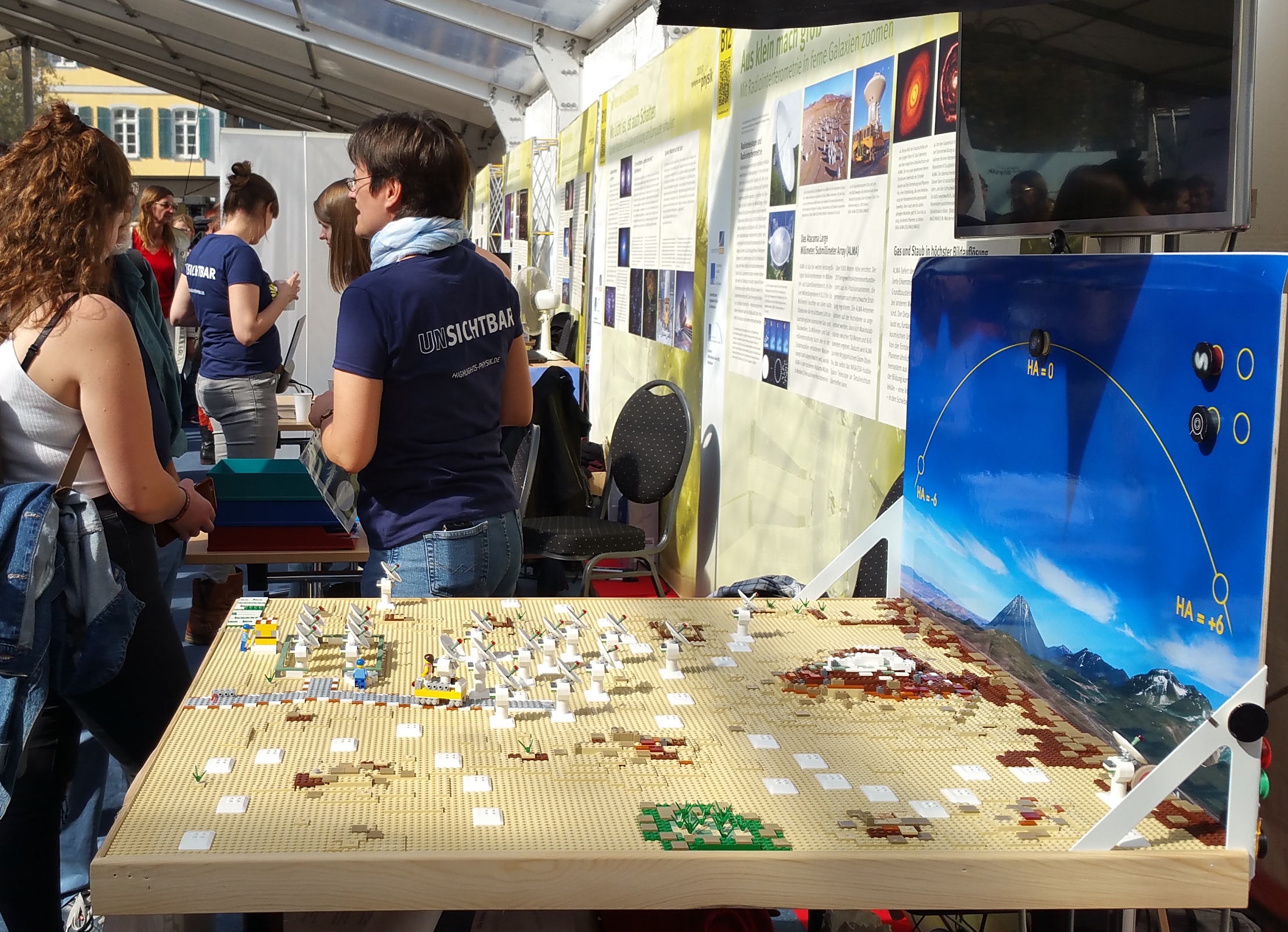
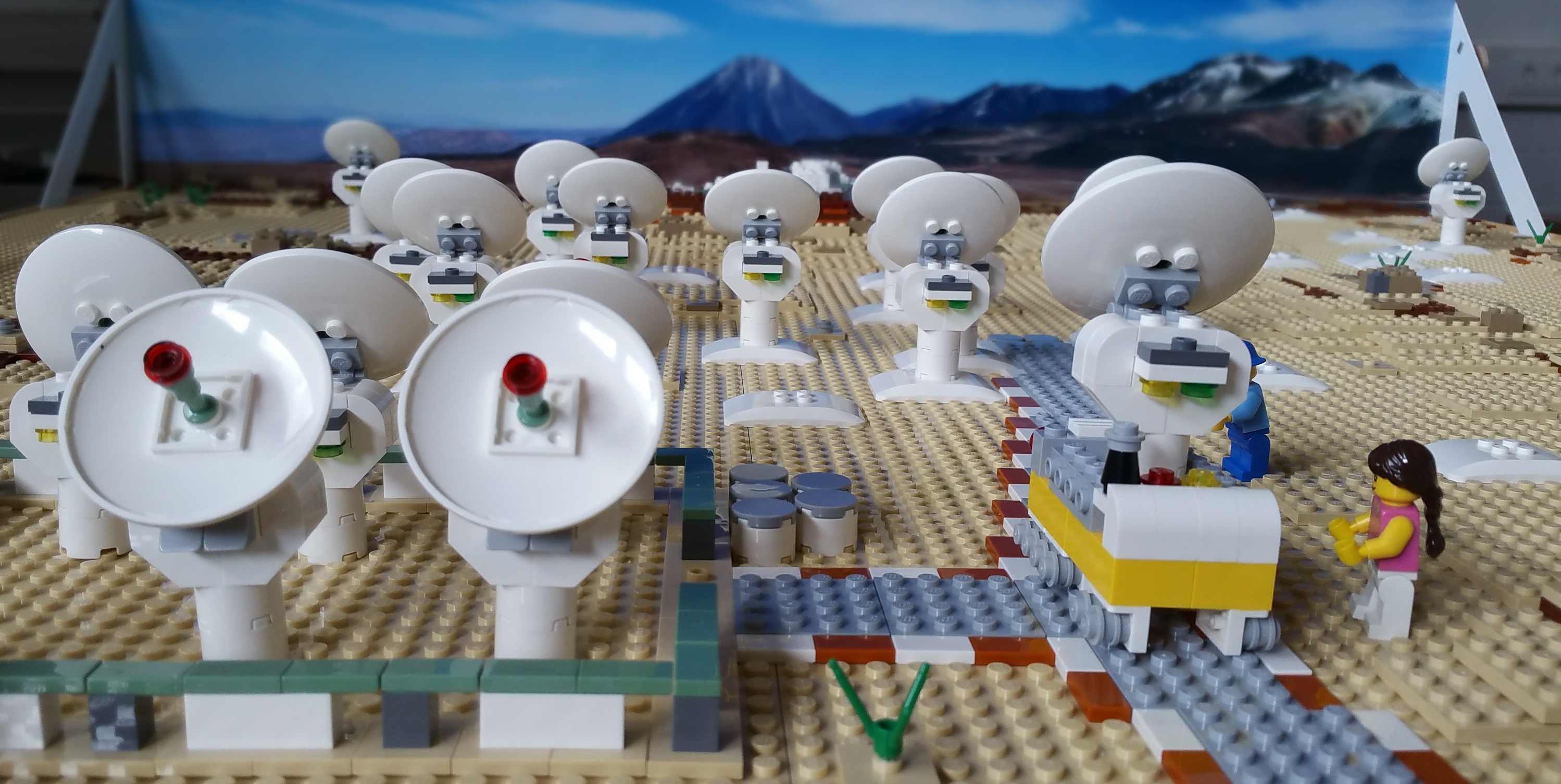
Die 19 LEGO-Teleskope können auf 43 vorbestimmte Positionen gesetzt werden. Das echte ALMA besitzt 66 Teleskope und 192 Positionen (pads) im maximalen Abstand von 16 km.
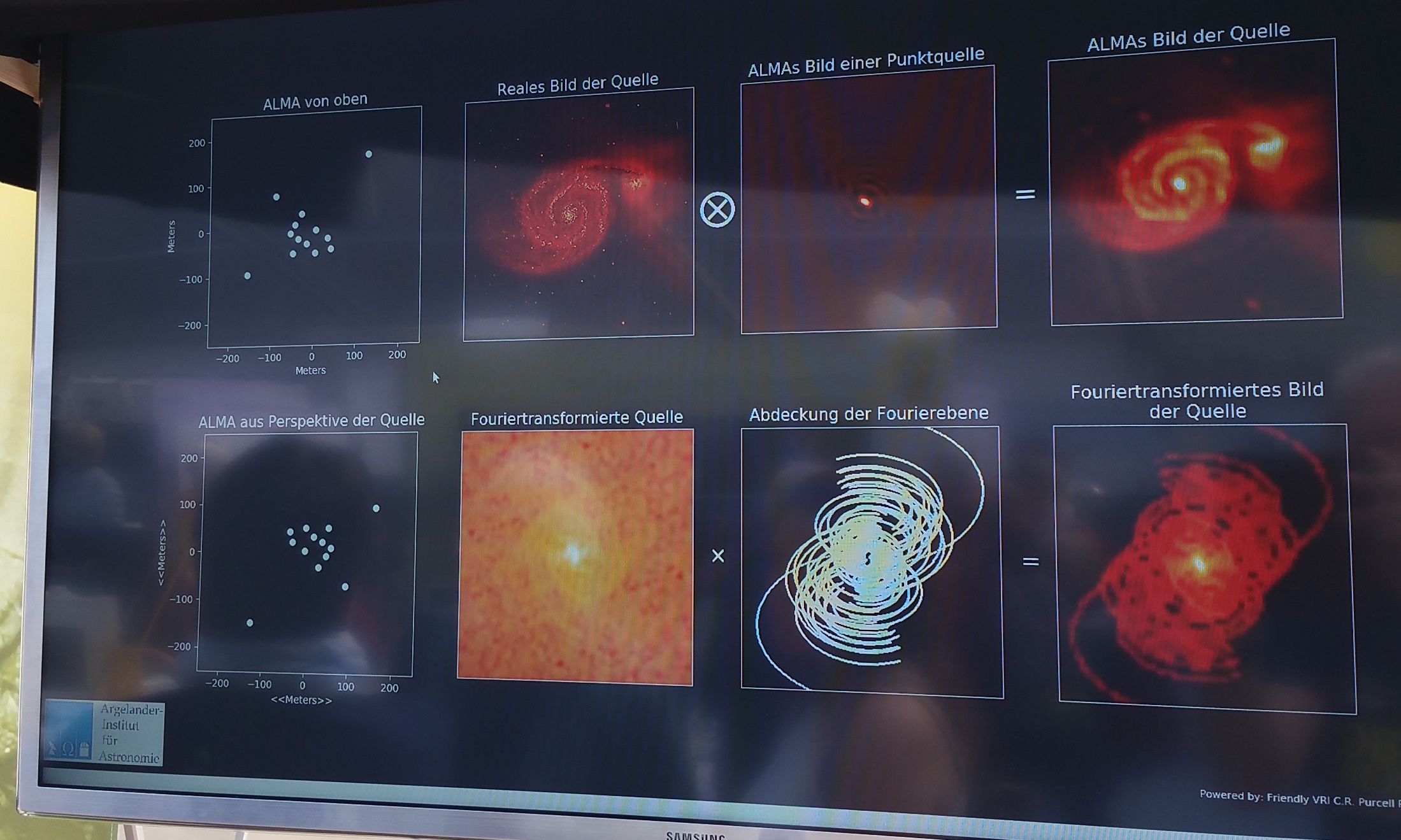
Auf dem Monitor werden verschiedene aktuelle Daten wiedergegeben: links oben die Positionen der Teleskope von oben gesehen, darunter aus Perspektive der Quelle zum Zeitpunkt ihres höchsten Standes; links oben mittig das reale Bild der Quelle, rechts oben das Bild, das ALMA mit der gewählten Konfiguration sehen würde wenn Daten über 12 Stunden aufgenommen würden (Auf- bis Untergang) und die Erddrehung dabei entsprechend die Perspektive der Teleskope dreht, daneben das Bild einer hypothetischen Punkt-quelle, dies entspricht dem Abbildungsvermögen dieser Beobachtung.
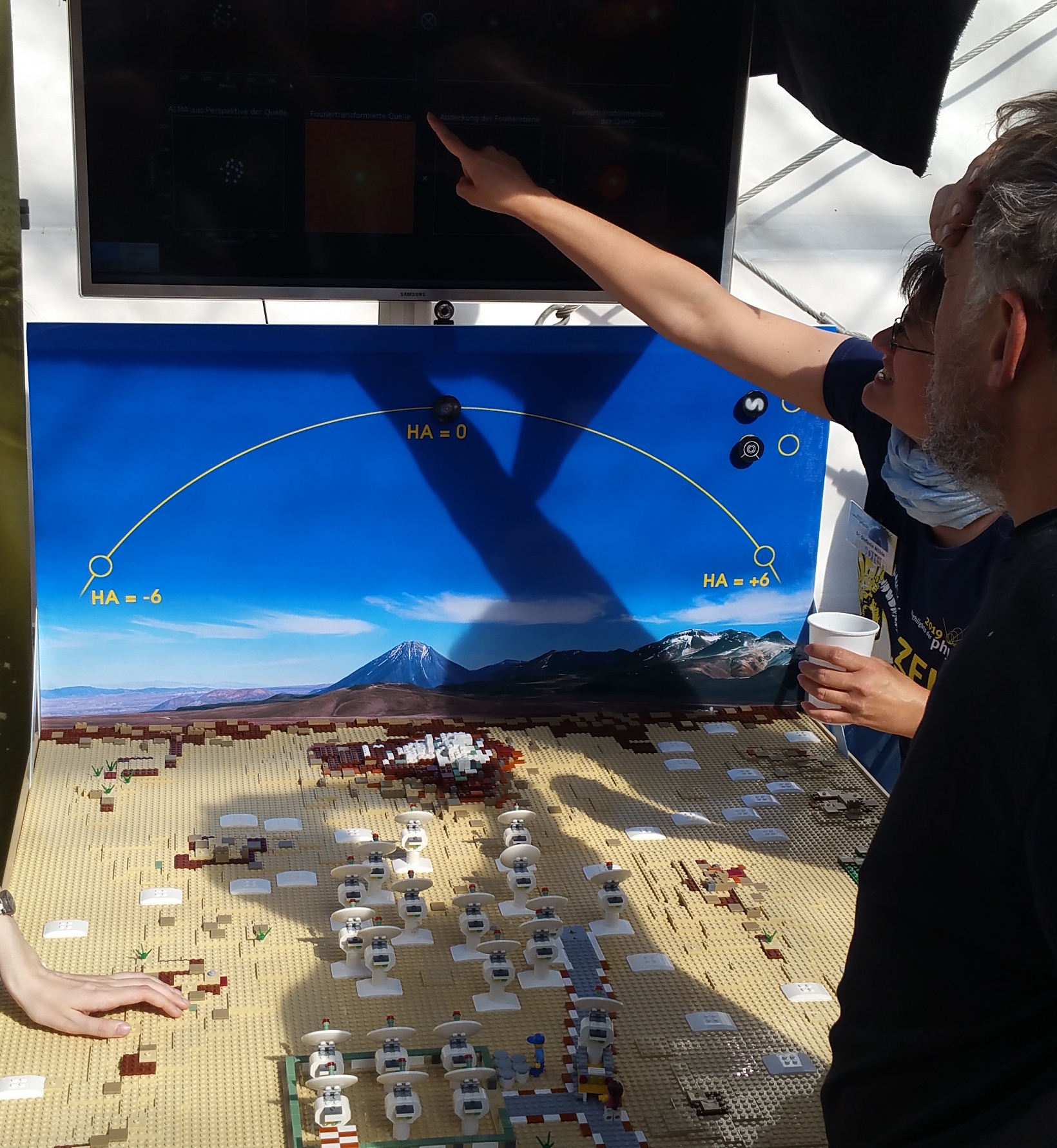
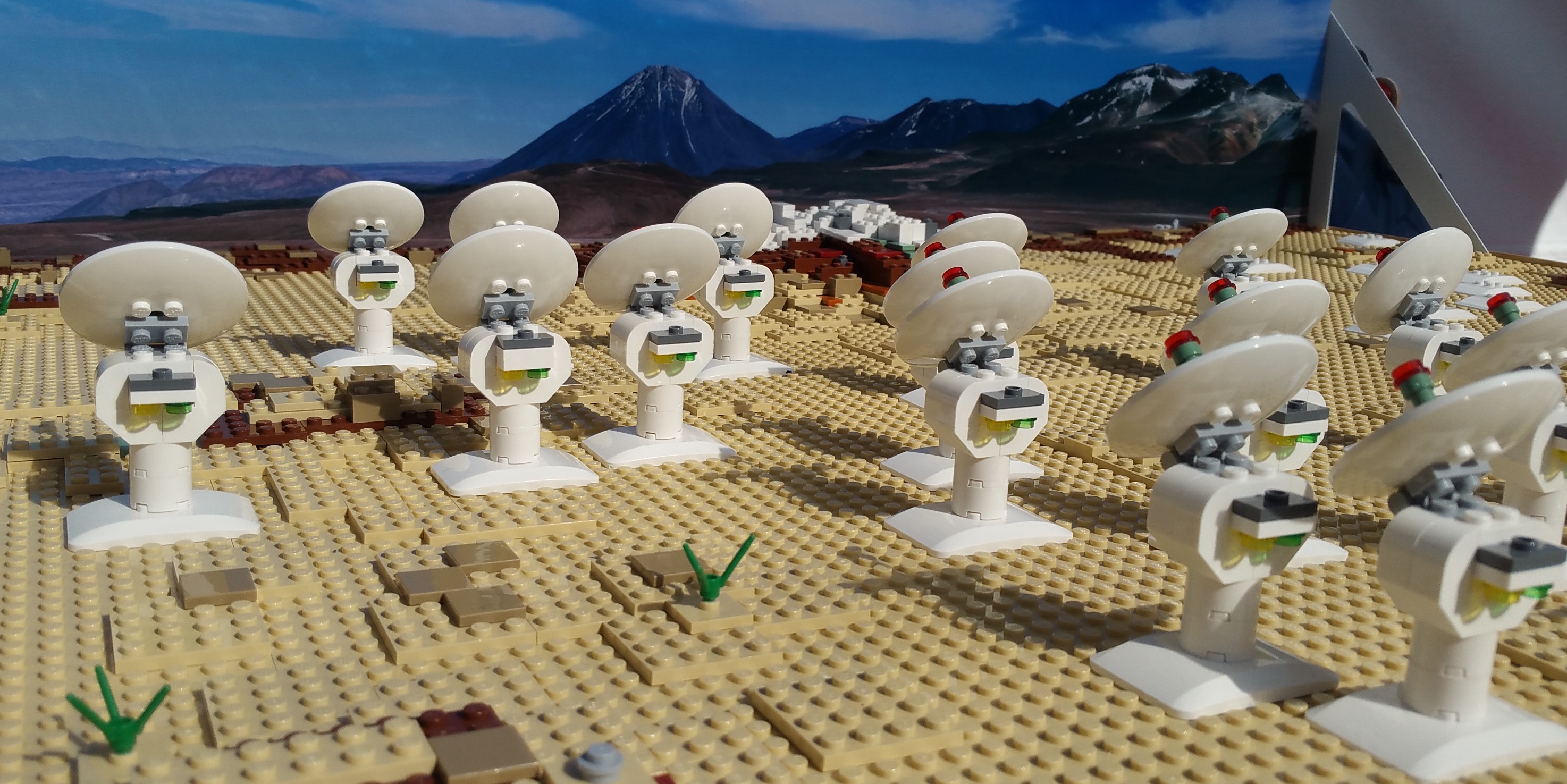